By Karen Hills
This is part of a series highlighting work by Washington State University (WSU) researchers through the Waste to Fuels Technology Partnership between the Department of Ecology and WSU during the 2017-2019 biennium.
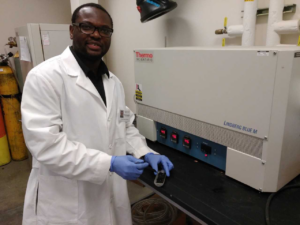
Biochar is produced by pyrolysis of woody (technically, lignocellulosic) materials. By controlling the conditions under which it is produced, researchers can engineer biochar to be more effective for particular purposes. In previous articles, I explored work looking at the potential for biochar to draw down atmospheric carbon dioxide and increase water holding capacity in soils. Michael Aniayia (Figure 1) and his colleagues in the lab of Dr. Manuel Garcia-Perez at Washington State University, engineered biochar for a specific purpose – adsorbing phosphate, a nutrient that, because it is also common in wastewater and manure, can pollute waterways. Aniayia’s objective was to evaluate strategies for producing biochar in order to improve its ability to remove phosphate.
Ayiania used and evaluated a number of strategies, each designed to change the physical or chemical makeup of the biochar to improve phosphate adsorption. He produced a first generation biochar using a pyrolysis step, followed by an activation step with carbon dioxide. Carbon dioxide-activated char from anaerobically digested fiber had phosphate adsorption capacity of 32.4 mg of phosphate per gram of biochar. He also produced a second generation biochar by “nitrogen doping.” Nitrogen doping is the process of introducing nitrogen functional groups into a carbonaceous material, like biochar. Nitrogen doping increased phosphate adsorption capacity to 110.3 mg of phosphate per gram of biochar. He also evaluated third generation biochar, produced by first impregnating the feedstock with metals (magnesium, calcium, or iron) and then using the N-doping process to create a metal-N-doped biochar. The synergy between magnesium and nitrogen doping resulted in biochar with an enhanced ability to adsorb phosphate (Figure 2). Biochar produced from cellulose that was magnesium-N-doped, had a phosphate adsorption capacity of 335 mg per gram of biochar – more than ten times the adsorption capacity of the first generation biochar.
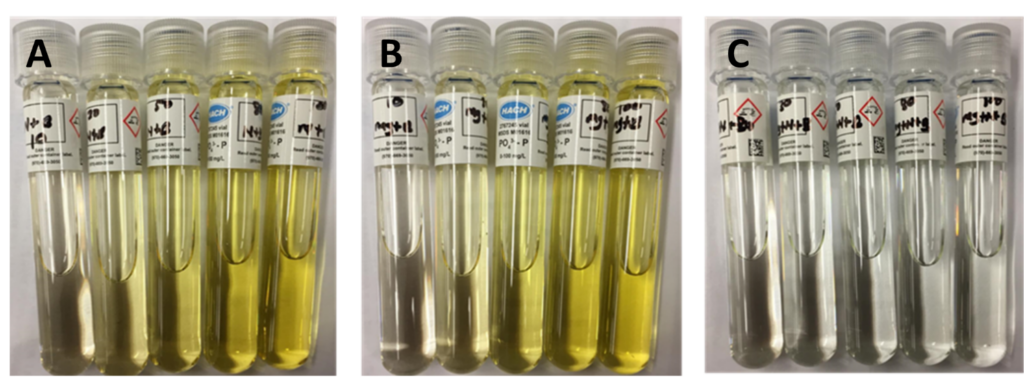
The bottom line is that Ayiania and colleagues were able to dramatically improve the capacity of biochar to adsorb phosphate. “Boutique biochars” like these, engineered for specific purposes, are an important specialty market for the biochar industry. From a climate change perspective, sizeable benefits will occur when large amounts of biochar are applied to the landscape, most likely as field-scale agricultural amendments. However, demand for biochar by agricultural producers is not widespread at its current price. Developing markets for high-value biochars could help support the emerging biochar industry. If economically feasible, they could also sequester small amounts of carbon as a side-benefit to their primary use, while helping agricultural producers become more familiar with these new materials. Further technological advances in the industry and policy changes (e.g., carbon policy) could lower cost of production and spur greater adoption by agriculture in the future, leading to carbon sequestration benefits.
For more detail, see the brief project report (7 pages, Chapter 7 in Hills et al. 2019) or the longer technical report (Ayiania et al., 2019: 71 pages).
References:
Ayiania, M., S. Haghighi Mood, Y.J. Milan, M. Garcia-Perez. 2019. Production of Engineered Biochars for Phosphate Removal from Waste Lignocellulosic Materials: First, Second, and Third Generation Engineered Products. A technical report completed as part of the Waste to Fuels Technology Partnership. 71 pp.
Hills, K., M. Garcia-Perez, J.E. Amonette, M. Brady, T. Jobson, D. Collins, D. Gang, E. Bronstad, M. Flury, S. Seefeldt, C.O. Stöckle, M. Ayiania, A. Berim, W. Hoashi-Erhardt, N. Khosravi, S. Haghighi Mood, R. Nelson, Y.J. Milan, N. Pickering, N. Stacey, A.H. Tanzil, J. Zhang, B. Saari, and G. Yorgey. Advancing Organics Management in Washington State: The Waste to Fuels Technology Partnership, 2017-2019 Biennium. 2019. Publication 19-07-027. Solid Waste Management Program, Washington Department of Ecology, Olympia, WA.
Comments